Precision in Practice: Mastering Lean Six Sigma
Journey with us into the world of Lean Six Sigma, where efficiency meets excellence. Uncover the secrets of process perfection.
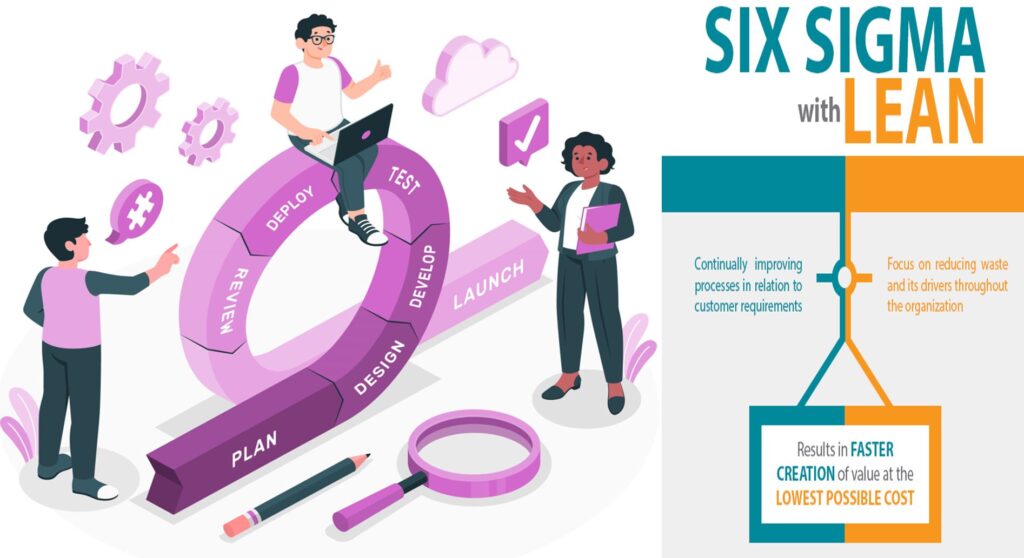
Quick Insights:
“Lean Six Sigma Unpacked: Quick and Effective Knowledge”
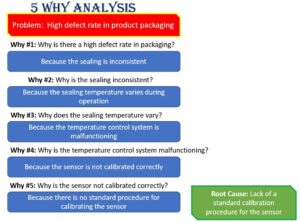
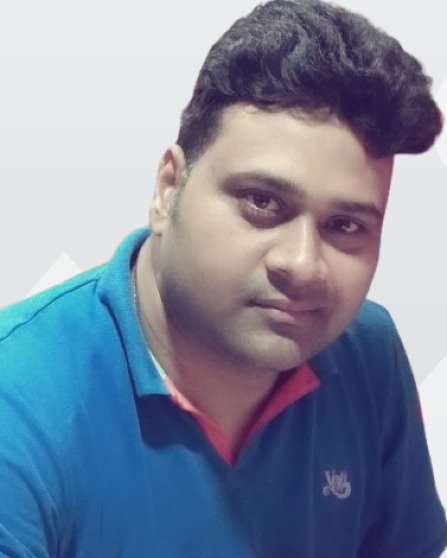
What is 5 Whys Analysis and how to use it?
Detailed Analysis of the 5 Whys RCA Method The 5 Whys is a simple yet powerful technique used in Root Cause Analysis (RCA) to drill
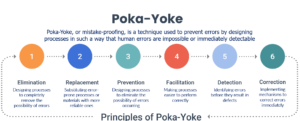
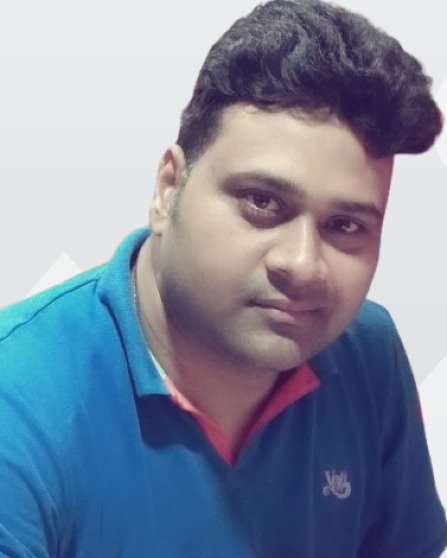
POKA YOKE Enhancing Quality with Foolproof Solutions
Introduction In the pursuit of operational excellence, minimizing errors and defects is a critical goal for any organization. One effective approach to achieving this is
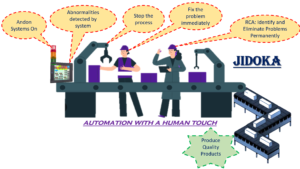
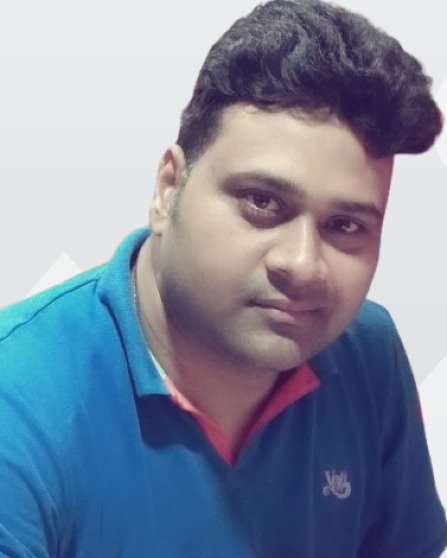
JIDOKA: Enhancing Efficiency with Smart Automation
Introduction In the realm of Lean Manufacturing, Jidoka stands out as a powerful concept that significantly enhances quality and efficiency. Often translated as “automation with
Deep Dives:
“In-Depth Lean Six Sigma Wisdom at Your Fingertips”
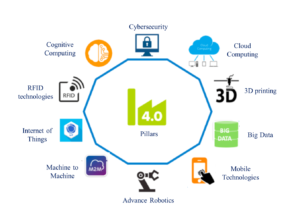
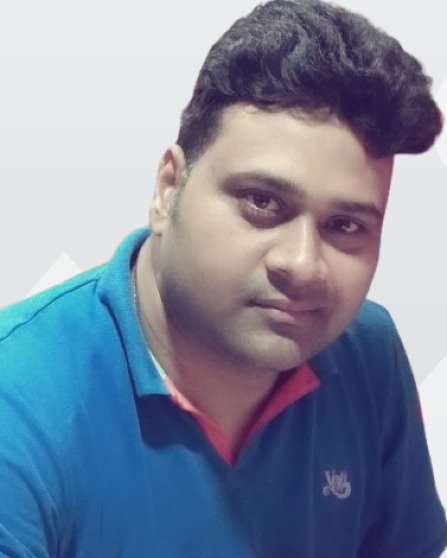
Discover Industry 4.0: How Lean Six Sigma Seamlessly Integrates for Maximum Efficiency
Introduction In the ever-evolving landscape of modern industries, the integration of Lean Six Sigma with Industry 4.0 stands as a transformative approach. By merging the
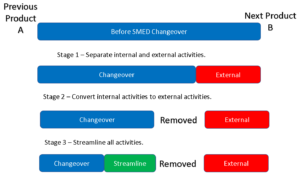
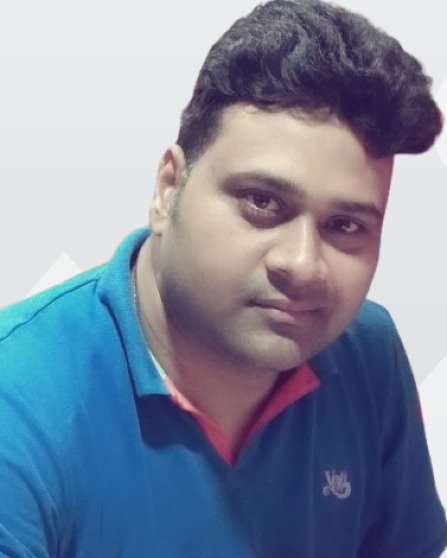
What is SMED?
What is SMED? SMED Definition: SMED stands for Single-Minute Exchange of Die. It is a Lean manufacturing technique aimed at reducing the setup or changeover time
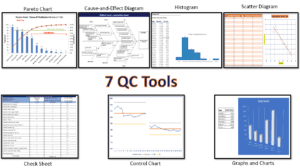
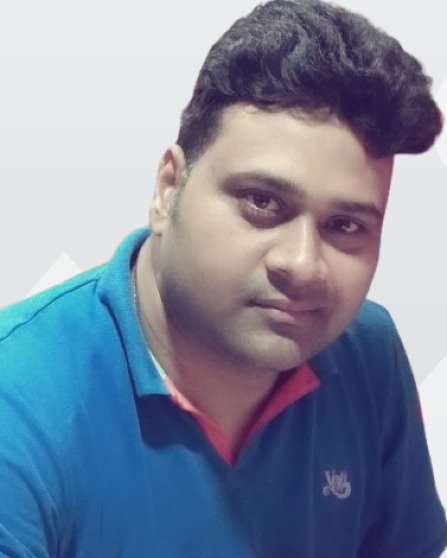
Revealing the Power of 7 QC Tools and Mastering Quality Excellence
Introduction: Quality control plays a crucial role in ensuring products and services meet the desired standards. It involves the systematic examination of processes to prevent
Pro Tips:
“Lean Six Sigma Insights in a Snap”
Lean Six Sigma is a data-driven approach to reduce the variation in the process and streamline it by eliminating waste.
Lean focuses on eliminating waste and optimizing process flow to enhance efficiency and reduce production time. It identifies and removes non-value-adding activities.
Six Sigma is a data-driven method that minimizes defects and variations in processes, resulting in higher quality and consistency. It relies on statistical analysis to make informed decisions.
Six Sigma is a methodology that seeks to achieve a high level of quality and process performance by reducing defects and variation. The quality levels of Six Sigma are typically expressed in terms of the number of defects per million opportunities (DPMO).
Here are the different quality levels within Six Sigma:
Six Sigma (6σ): This is the highest level of quality, with only 3.4 defects per million opportunities. It represents a process that operates with a high degree of precision and consistency.
Five Sigma (5σ): At this level, there are 233 defects per million opportunities. While it does not reach the same level of perfection as Six Sigma, it still signifies a very high-quality process.
Four Sigma (4σ): This level has 6,210 defects per million opportunities. It indicates a process that is reasonably good but has room for improvement.
Three Sigma (3σ): With 66,807 defects per million opportunities, this level represents a process with moderate quality but noticeable variations and defects.
Two Sigma (2σ): At this level, there are 308,537 defects per million opportunities. It signifies a process that is often unreliable and inefficient.
One Sigma (1σ): This is the lowest level of quality, with 691,462 defects per million opportunities. It represents a process that is highly unreliable and produces a significant number of defects.
In Lean Six Sigma, USL (Upper Specification Limit) represents the highest acceptable value for a product or process, while LSL (Lower Specification Limit) is the lowest acceptable value. The “Target” is the ideal or desired value you aim to achieve. These three parameters help define the acceptable range for a product’s quality, with the goal of keeping the process within these limits to meet customer requirements and minimize defects.
CTQ, or Critical to Quality, is a fundamental concept in Lean Six Sigma. It refers to the specific aspects or characteristics of a product or process that are crucial to meeting customer requirements and expectations. Identifying and focusing on CTQs is essential for delivering quality and ensuring customer satisfaction. By understanding what is critical to quality, Lean Six Sigma practitioners can target their efforts to improve these key attributes and, in turn, enhance overall product or process performance.
Examples of CTQs (Critical to Quality) in different contexts:
Pharmaceutical Tablet Manufacturing:
CTQ: Dissolution Rate
Explanation: In pharmaceutical tablet manufacturing, the dissolution rate of a tablet in a patient’s body is critical to quality. This parameter determines how quickly and completely the tablet disintegrates and releases its active ingredients. The dissolution rate directly affects the effectiveness of the medication. Ensuring that tablets consistently meet the specified dissolution rate is vital for pharmaceutical quality control and patient safety.
Bottling Line in a Soft Drink Factory:
CTQ: Fill Level Accuracy
Explanation: In a soft drink bottling line, the fill level of each bottle is critical to quality. Customers expect the right amount of product in each bottle. Deviations from this standard can result in customer dissatisfaction and potential legal issues.
Online Retail Order Fulfillment:
CTQ: Order Delivery Time
Explanation: For an online retail business, the time it takes to deliver orders to customers is critical to quality. Prompt delivery is a key customer expectation, and delays can lead to customer complaints and loss of business.
Hospital Emergency Room Operations:
CTQ: Patient Wait Time
Explanation: In a hospital’s emergency room, the time a patient has to wait for initial assessment and treatment is critical to quality. Reducing wait times is essential for patient care, as prolonged delays can impact patient outcomes and satisfaction.
In Lean Six Sigma, a “defect” refers to any deviation or error in a product or process that does not meet the defined quality standards or customer expectations. It is an event that does not meet at least one of the specified CTQs. It can manifest as a flaw, non-conformance, or any undesirable characteristic that impacts the product’s performance, appearance, or functionality. Identifying and addressing defects is a core objective of Lean Six Sigma, aiming to improve quality, reduce waste, and enhance customer satisfaction.
Examples of defects across various industries:
Manufacturing – Automotive Industry:
Scratches on Car Body: Visible scratches on the car’s exterior surface that affect its appearance.
Software Development:
Bugs in Software Code: Programming errors that cause software applications to malfunction or crash.
Healthcare – Radiology Imaging:
Misinterpretation of X-Rays: An incorrect diagnosis or failure to identify a medical condition in an X-ray image.
Food Production – Bakery:
Burnt or Overbaked Bread: Baking bread beyond the optimal time, resulting in a burnt or overly crispy texture.
Construction:
Cracks in Building Foundation: Structural cracks in a building’s foundation that can compromise its integrity.
Call Center – Customer Service:
Incorrect Information Provided: Giving customers incorrect information or resolving issues inaccurately.
Textile Industry:
Torn Fabric: Fabric that has rips, tears, or holes due to manufacturing or handling issues.
Pharmaceutical Manufacturing:
Contaminated Medication: Medications that contain impurities, foreign particles, or incorrect ingredients.
In Lean Six Sigma, an “opportunity” refers to a chance for a defect or error to occur in a process. It’s the specific point in a process where a product or service could fail to meet customer requirements or quality standards. Understanding and accurately defining opportunities is crucial for calculating process performance and identifying areas for improvement. It helps Lean Six Sigma practitioners focus their efforts on preventing defects at these critical points in a process.
Defects-per-unit (DPU) is a key performance metric used to measure the average number of defects in a single unit of a product or service. It provides a clear and quantifiable picture of quality issues and is essential for identifying areas for improvement. Calculating and monitoring DPU is a fundamental step in Lean Six Sigma, helping organizations enhance quality, reduce defects, and meet customer expectations effectively.
Example: you are working in a manufacturing facility that produces electronic components. Your team is responsible for inspecting circuit boards for defects. Over the course of a week, you have inspected 500 circuit boards and found various defects. You want to calculate the DPU to assess the quality of your process.
Total number of circuit boards inspected: 500
Total number of defects found: 30
DPU = (Total Defects) / (Total Units)
DPU = 30 / 500
DPU = 0.06 defects per unit
Defects-per-Opportunity (DPO) is a crucial metric in Lean Six Sigma used to measure the number of defects in a process relative to the number of opportunities for defects to occur. It provides a detailed view of quality performance, helping organizations identify areas for improvement. Calculating DPO enables you to assess and enhance the quality of your processes and products by focusing on the specific opportunities for defects.
Example: For a data entry process where you are inputting customer information into a database. Each customer record has 5 fields, and there are multiple opportunities for data entry errors. Over a week, you have processed 1,000 customer records and found a total of 200 data entry errors.
Total number of customer records processed: 1,000
Total number of data entry errors found: 200
DPO = (Total Defects) / (Total Opportunities)
DPO = 200 / (1,000 records * number of fields with data entry opportunities)
For each customer record, there are 5 fields with data entry opportunities:
DPO = 200 / (1,000 * 5) = 0.04 DPO
In this example, the DPO is 0.04, which means there are, on average, 0.04 defects for each data entry opportunity (field in this case).
Defects-per-million opportunities (DPMO) is a critical Lean Six Sigma metric that quantifies the number of defects in a process for every one million opportunities. It is a valuable measure for assessing process quality and performance. DPMO helps organizations understand the extent of defects in their processes and sets a standard for improving quality. Calculating DPMO is a fundamental step in Lean Six Sigma, focusing on minimizing defects and enhancing customer satisfaction.
Refer DPO Example for DPO value:
DPO = 0.04 (from the data entry process example)
Refer DPU Example for DPU value:
DPU = 0.06 (from the electronic components manufacturing example)
To calculate DPMO, we use the following formula:
DPMO = DPO (or DPU) * 1,000,000
DPO-based DPMO:
DPMO = 0.04 * 1,000,000 = 40,000 DPMO
DPU-based DPMO:
DPMO = 0.06 * 1,000,000 = 60,000 DPMO
In these examples, the DPMO for the data entry process is 40,000, and the DPMO for electronic component manufacturing is 60,000. These values indicate the number of defects per one million opportunities in their respective processes. Lower DPMO values signify better process quality and fewer defects.
The Cost of Quality (COQ) in Lean Six Sigma represents the total expenses associated with ensuring the quality of products or services. It includes both the cost of preventing defects (prevention costs) and the cost of dealing with defects that occur (appraisal and failure costs).
Prevention Costs:
Definition: Prevention costs are incurred to prevent defects from occurring in the first place. These costs are associated with activities and investments that ensure processes are designed, executed, and maintained effectively to minimize the likelihood of defects.
Examples: Quality planning, employee training, process design, and supplier quality control. These activities help prevent defects from entering the production process.
Appraisal Costs:
Definition: Appraisal costs are associated with the inspection and evaluation of products or services to ensure they meet quality standards. These costs occur after the production process to detect and correct defects.
Examples: Inspection, testing, and quality audits. Appraisal activities help identify defects before products reach customers.
Internal Failure Costs:
Definition: Internal failure costs are incurred when defects are detected within the organization before products or services are delivered to customers. These costs are associated with correcting defects, rework, and scrap.
Examples: Rework, scrap, machine downtime due to defects, and retesting. Internal failure costs address defects found during the production process.
External Failure Costs:
Definition: External failure costs are incurred when defects are discovered by customers after they have received products or services. These costs can be the most damaging to a company’s reputation and finances.
Examples: Warranty claims, customer returns, product recalls, and legal costs. External failure costs relate to defects found in the hands of customers.
Calculating COQ:
COQ is calculated by summing the costs from each of these four categories:
COQ = Prevention Costs + Appraisal Costs + Internal Failure Costs + External Failure Costs
Understanding COQ is crucial for organizations aiming to improve quality and reduce waste. By analyzing and minimizing these costs, businesses can enhance their overall profitability, customer satisfaction, and reputation while delivering higher-quality products and services. Lean Six Sigma practitioners use COQ to identify areas for improvement and prioritize projects that yield the most significant cost and quality benefits.
Example: COQ in a Widget Manufacturing Company
Suppose you manage a company that manufactures widgets. You’re interested in understanding the Cost of Quality to identify opportunities for cost reduction and process improvement.
Prevention Costs:
Quality Training: You invest $10,000 in training programs for your staff to ensure they produce widgets correctly.
Quality Planning: You spend $5,000 on quality planning and process design.
Appraisal Costs:
Inspection: You conduct product inspections costing $8,000 to check the quality of widgets before they’re shipped.
Internal Failure Costs:
Rework: You spend $6,000 on fixing defects found during the manufacturing process.
Scrapped Widgets: Some widgets are so defective that you have to scrap them, costing $2,000.
External Failure Costs:
Customer Returns: Customers return defective widgets, resulting in a $4,000 cost.
Warranty Claims: You pay $3,000 in warranty claims for widgets that failed in the hands of customers.
Now, let’s calculate the Cost of Quality:
Total Prevention Costs: $10,000 (Training) + $5,000 (Planning) = $15,000
Total Appraisal Costs: $8,000 (Inspection)
Total Internal Failure Costs: $6,000 (Rework) + $2,000 (Scrapped) = $8,000
Total External Failure Costs: $4,000 (Returns) + $3,000 (Warranty Claims) = $7,000
Total COQ: $15,000 (Prevention) + $8,000 (Appraisal) + $8,000 (Internal Failure) + $7,000 (External Failure) = $38,000
In this example, the Cost of Quality for widget manufacturing is $38,000. By breaking down these costs, you can identify areas for improvement and cost reduction, ultimately enhancing the quality of your widgets and the profitability of your business.
The Cost of Poor Quality (COPQ) is the total expenses incurred by a business due to the production of products or services that do not meet quality standards. This includes the costs associated with defects, rework, warranty claims, customer complaints, and any other quality-related issues. COPQ represents the financial impact of not delivering products or services that meet customer expectations and industry standards, and it encompasses both the visible, direct costs and the hidden, indirect costs associated with poor quality. Reducing COPQ is a significant focus in quality management, as it can significantly impact a company’s profitability and reputation.
DMADV, which stands for Define, Measure, Analyze, Design, and Verify, is a structured problem-solving methodology within Lean Six Sigma. It is used to develop new processes or products that meet or exceed customer expectations. DMADV ensures that the final output is of the highest quality and precisely aligned with customer requirements, making it a valuable tool in process improvement and innovation.
SMED, or Single-Minute Exchange of Die, is a Lean Six Sigma methodology aimed at minimizing the time required for equipment setup and changeovers in manufacturing processes. The term “single-minute” may be somewhat misleading; it signifies the goal of reducing changeover times to the smallest practical duration, not necessarily one minute.
SMED is especially beneficial in industries where frequent equipment changeovers are necessary, such as automotive manufacturing or food processing. It focuses on separating internal (tasks that can only be done when the machine is stopped) and external (tasks that can be done while the machine is running) setup activities. By optimizing and simplifying these activities, SMED reduces downtime, enhances production flexibility, and ultimately boosts productivity.
The process typically involves:
Documentation: Detailed recording of the current setup process, which helps identify bottlenecks and areas for improvement.
Separation: Distinguishing internal and external setup tasks to make adjustments that can be done without stopping the machine.
Conversion: Transforming internal setup tasks into external setup tasks where possible.
Streamlining: Simplifying both internal and external setup tasks to minimize the time required for each.
Standardization: Establishing best practices and standardized procedures for future setups.
SMED not only benefits manufacturing by increasing efficiency but also reduces costs associated with wasted time and resources during changeovers. It allows companies to be more agile, responding promptly to customer demands and variations in production requirements.
ECRS, an acronym in Lean Six Sigma methodology, stands for Eliminate, Combine, Rearrange, and Simplify. It is a systematic approach used to optimize processes by identifying inefficiencies and streamlining operations for improved efficiency and effectiveness. ECRS is used to find out the unnecessary/complex activities and work on them to improve the process.
Eliminate: This step involves identifying and eliminating unnecessary or non-value-added activities, processes, or steps. By removing waste, organizations can streamline their processes and focus resources on activities that add value to the customer.
Combine: In this phase, similar or redundant activities are combined to reduce complexity and improve efficiency. By consolidating tasks, organizations can eliminate duplication of efforts and resources, leading to streamlined processes.
Rearrange: Rearranging involves reorganizing the sequence or layout of activities to optimize workflow and minimize unnecessary movement or transportation. By rearranging processes, organizations can reduce lead times and improve overall productivity.
Simplify: Simplification focuses on making processes easier to understand, execute, and manage. This may involve standardizing procedures, reducing unnecessary complexity, or providing training to improve employee proficiency. Simplified processes are more efficient, less error-prone, and easier to sustain.
By applying ECRS principles, organizations can identify opportunities for improvement, streamline processes, and achieve operational excellence in Lean Six Sigma initiatives.
In Lean Six Sigma, VA stands for Value-Added, BVA stands for Business value-added, and NVA stands for Non-Value-Added. These terms are used to differentiate between activities that directly contribute to meeting customer needs and those that do not.
Value-Added (VA): Value-Added activities are those that directly contribute to transforming a product or service in a way that the customer is willing to pay for. These activities add value to the end product or service and are essential for meeting customer requirements.
Example: In a manufacturing process, value-added activities can include: receiving a part request, preparing an internal request for a part from production, finding production availability, updating part request information, packaging and the manager processing the part request
Non-Value-Added (NVA): Non-Value-Added activities are those that do not contribute to meeting customer needs or enhancing the quality of the product or service. These activities are considered wasteful and should be minimized or eliminated from the process to improve efficiency and reduce costs.
Example: Waiting time between process steps, such as idle time on a production line, is a non-value-added activity as it does not contribute to the production process but increases lead time and wastes resources.
Excess inventory storage, where products sit unused for extended periods, is another example of NVA, as it ties up capital and space without providing any value to the customer.
Business Value Added (BVA): Business Value Added refers to any aspect of a business process that is essential for its operation and survival but does not directly contribute to the creation of the final product or service, nor is it directly valued by customers. These activities are necessary to maintain the business’s operations but do not enhance the product or service in the eyes of the customer.
Example: administrative tasks such as accounting, payroll, or regulatory compliance may be considered BVA because they are necessary for the business to function smoothly, yet they do not directly impact the product or service offered to customers. Investing in employee training programs to enhance skills and knowledge contributes to BVA by improving overall productivity, quality, and customer satisfaction. Implementing sustainable practices in manufacturing processes, such as energy-efficient equipment or waste reduction initiatives, adds BVA by reducing costs, improving environmental sustainability, and enhancing brand reputation.
Identifying and minimizing BVA is a key focus of Lean Six Sigma methodologies, as it allows organizations to streamline their processes, reduce waste, and allocate resources more efficiently, ultimately improving overall productivity and profitability.
Identifying and eliminating NVA activities while maximizing VA activities is a fundamental principle of Lean Six Sigma. By focusing on value-added activities, organizations can streamline their processes, reduce waste, and improve overall efficiency and customer satisfaction.
Total Productive Maintenance (TPM) is a comprehensive approach to equipment maintenance that aims to maximize the effectiveness of production equipment while involving all employees in the maintenance process. The goal of TPM is to achieve zero breakdowns, zero defects, and zero accidents by focusing on proactive and preventive maintenance practices. TPM emphasizes a holistic approach to maintenance that addresses the entire lifecycle of equipment, from installation to disposal, and encourages collaboration between production operators and maintenance personnel. By implementing TPM, organizations can improve equipment reliability, increase productivity, reduce costs, and create a safer work environment.
- Autonomous Maintenance (Jishu Hozen): Involves empowering operators to take responsibility for routine equipment maintenance tasks, such as cleaning, inspection, lubrication, and minor repairs. By actively involving operators in the care of equipment, organizations can improve equipment reliability and identify issues early.
- Planned Maintenance: Focuses on scheduling and conducting preventive maintenance activities based on equipment performance data, manufacturer recommendations, and predictive maintenance techniques. Planned maintenance helps minimize unplanned downtime, extend equipment life, and optimize maintenance resources.
- Focused Improvement (Kobetsu kaizen): Encourages continuous improvement efforts aimed at eliminating losses, improving equipment efficiency, and enhancing overall productivity. Focused improvement initiatives typically involve cross-functional teams identifying and addressing the root causes of equipment-related issues.
- Early Equipment Management: This involves considering maintenance requirements and reliability concerns during the design and installation of new equipment or processes. By proactively addressing maintenance considerations upfront, organizations can reduce future maintenance requirements and improve equipment reliability.
- Education and Training: Ensures that employees at all levels have the necessary skills and knowledge to effectively participate in TPM activities. Education and training programs cover topics such as equipment operation, maintenance procedures, problem-solving techniques, and TPM principles.
- Quality Maintenance: Focuses on preventing defects and ensuring product quality by addressing equipment-related issues that could lead to quality issues or production downtime. Quality maintenance activities aim to maintain equipment in optimal condition to produce defect-free products consistently.
- Safety, Health, and Environment: Prioritizes the safety and well-being of employees, as well as environmental sustainability, throughout the TPM process. Safety, health, and environmental considerations are integrated into all TPM activities to create a safe and sustainable work environment.
These pillars provide a framework for organizations to systematically improve equipment reliability, productivity, and overall performance through the implementation of TPM principles and practices.
In Autonomous Maintenance, an abnormality refers to any deviation from the normal condition of equipment during operation, indicating potential issues with performance, functionality, or reliability. These abnormalities can include unusual sounds, vibrations, visual defects, or any other irregularities that suggest the equipment is not operating optimally. Identifying and addressing abnormalities promptly is crucial for preventing equipment failures, ensuring smooth operations, and maintaining overall equipment effectiveness.
In Autonomous Maintenance, deterioration refers to the gradual decline in equipment condition over time, resulting in reduced performance, reliability, and efficiency. There are two types of deterioration: natural and force deterioration.
Natural deterioration occurs when the life of parts or equipment ends naturally due to wear and tear or aging. On the other hand, force deterioration happens when we accelerate the failure of parts or equipment before their natural lifespan by neglecting basic maintenance and operating conditions.
Reasons for force deterioration include:
- Poor cleaning of the machine, leads to the buildup of dirt, debris, and contaminants that can cause damage and reduce performance.
- Improper or lack of lubrication can result in increased friction, wear, and heat generation, leading to premature failure of moving parts.
- Looseness of machine parts is due to improper installation or maintenance, causing misalignment, vibration, and accelerated wear.
- Not maintaining the operating condition of the machine, such as operating it outside its specified parameters or overloading it, leads to stress, fatigue, and eventual failure of components.
Forced deterioration of parts can be avoided by maintaining the basic condition and operating condition of the machine.
Addressing force deterioration requires proactive maintenance practices, including regular cleaning, proper lubrication, tightening of loose parts, and adherence to operating guidelines and conditions. By addressing these factors, organizations can extend the life of their equipment, improve reliability, and optimize overall equipment performance.
This table highlights the key differences and similarities between SMED and ECRS, providing a clear comparison of their focus, objectives, applications, methodologies, and outcomes.
Aspect | ||
Focus | Reducing setup or changeover times in manufacturing | Broad process improvement across various industries |
Objective | Enable quick transitions between production runs (under 10 minutes) | Optimize processes by eliminating unnecessary steps and simplifying |
Application | Manufacturing environments | Any process in any industry |
Scope | Specific to changeover processes | Broad application across multiple processes |
Key Elements | 1. Identify Internal and External Activities | 1. Eliminate |
2. Convert Internal to External Activities | 2. Combine | |
3. Streamline External Activities | 3. Rearrange | |
4. Implement Quick Changeover Techniques | 4. Simplify | |
5. Test and Refine |
| |
6. Document and Standardize | ||
Methodology | Focus on distinguishing internal and external activities, then optimizing | Sequential approach to eliminate, combine, rearrange, and simplify |
Outcome | Faster changeovers, reduced downtime, increased production flexibility | Streamlined and efficient processes, higher productivity, reduced costs |
PDCA (Plan-Do-Check-Act) is a continuous improvement cycle used in business process management and Lean methodologies. It provides a structured framework for problem-solving and process improvement in a systematic way. Here’s a breakdown of the four stages:
- Plan: In this phase, the problem or opportunity for improvement is identified, and the root cause is analyzed. Clear objectives are set, and a detailed action plan is developed. This involves defining what needs to be changed, setting goals, and establishing metrics for success.
- Do: Once the plan is in place, the next step is to implement the solution or changes on a small scale. This is often done through pilot projects or testing to observe how the planned changes affect the process and to ensure the new method works as intended.
- Check: In this stage, the results of the implemented changes are measured and analyzed against the goals set in the planning phase. This step helps to verify if the new process is successful or if there are any discrepancies that need to be addressed.
- Act: Based on the findings from the “Check” phase, the process is either fully implemented if successful or adjustments are made for further improvement. If the plan didn’t work as expected, the cycle begins again with a revised approach.
How PDCA Works:
PDCA is a cyclical model, meaning it’s an ongoing process. Once improvements are made, the cycle starts again, focusing on new challenges and refining existing processes. This method is central to the Lean and Six Sigma methodologies, helping organizations to continually improve their efficiency, quality, and overall performance.
RCA (Root Cause Analysis) is a method used to identify the underlying cause or causes of a problem, defect, or failure. Instead of addressing the symptoms, RCA focuses on finding the fundamental issue to prevent the problem from recurring. It is widely used in Lean Six Sigma and other quality management methodologies to drive continuous improvement.
Key Steps in Root Cause Analysis:
- Define the Problem: Clearly identify and describe the issue, including the symptoms and impact on the process or system. This sets the foundation for a focused investigation.
- Collect Data: Gather relevant data to understand the full scope of the problem, including when and where it occurred, as well as the conditions leading up to it.
- Identify Possible Causes: Use tools like the 5 Whys, Failure Mode and Effects Analysis (FMEA), Pareto Analysis, Fault Tree Analysis (FTA), Scatter Diagrams, Fishbone (Ishikawa) Diagrams, or brainstorming sessions to generate potential causes. These tools help to dig deeper into the contributing factors.
- Analyse the Root Cause: Evaluate the possible causes identified and determine the true root cause(s). This might involve further investigation, testing, or validating hypotheses to ensure accuracy.
- Implement Corrective Actions: Once the root cause is confirmed, design and implement a solution to address it directly. Ensure that the action is sustainable and prevents recurrence.
- Monitor and Evaluate: After the solution is implemented, monitor the process to verify if the problem is resolved. Continue observing to ensure the issue does not reappear over time.
Importance of RCA:
- Prevention: RCA is designed to fix problems at their source, preventing them from happening again.
- Improved Efficiency: By addressing root causes rather than symptoms, RCA leads to more effective problem-solving and reduces the likelihood of recurring issues.
- Sustainability: Corrective actions derived from RCA ensure long-term improvements, enhancing the reliability of systems and processes.
RCA is a critical part of the continuous improvement process, particularly in industries focused on quality control, manufacturing, healthcare, and safety.